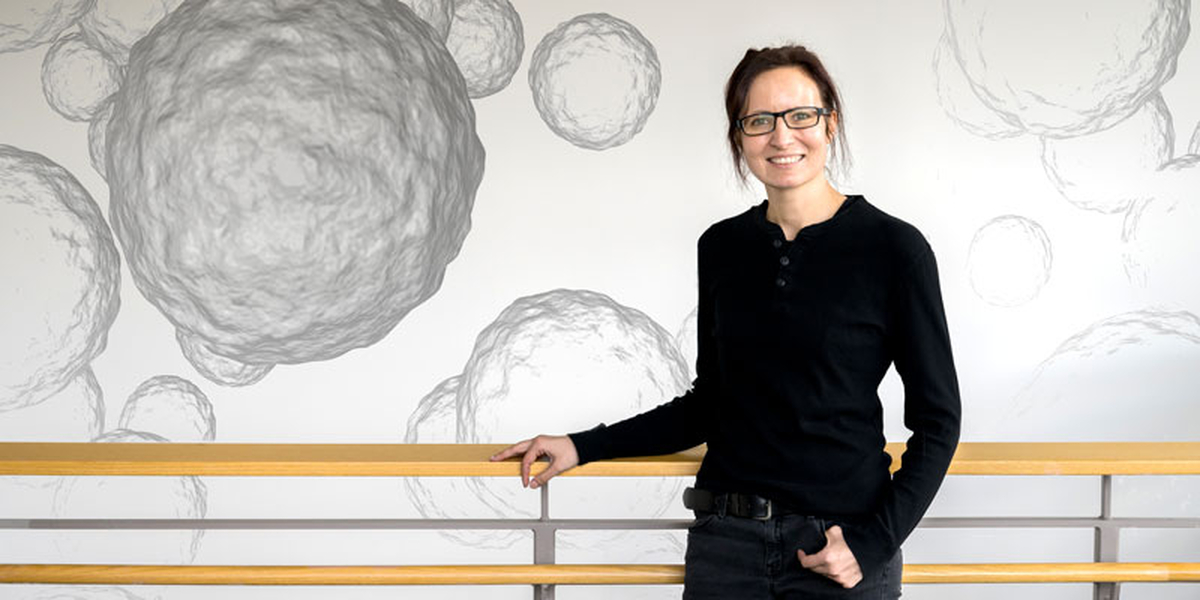
An astronaut battles a grueling headache. The pain reliever they brought on their mission is gone. Luckily, the ship is outfitted with an onboard mini-pharmaceutical factory powered by a synthetic cell system that can crank out a pill within a few hours.
It might sound a little like a science fiction novel, but Kate Adamala, an assistant professor in the Department of Genetics, Cell Biology, and Development, believes the scene is not that far off. Scientists expect to leverage synthetic cells to produce chemicals used in pharmaceutical drugs. The implications are farreaching for life in space and here on Earth.
“When I was a kid I learned about astrobiology from science fiction movies and I thought it was so cool,” says Adamala. “Then I realized it was something I could do for a living.” Now, Adamala is part of a small but growing field of researchers working to create a programmable and controllable synthetic cell system.
“The research I do is like science fiction with real-life applications,” says Adamala. “It’s the best of both worlds.”
Life, mirrored
Cells function as factories, power plants, transportation networks, and warehouses. They’re the smallest unit of life and they self-replicate, self-heal, and self-destruct. They carry out a wide variety of specialized functions thanks to the constant pressures of evolution and time.
Despite their incredible complexity, they’re messy. Like some basements, there is a lot of unnecessary junk that’s accumulated over a few decades (for cells, billions of years). Evolution does not act with a grand plan or end goal, which often leaves engineers and biologists scratching their heads at the inefficiencies of biological systems.
One of the starkest examples of this in cell systems is visible in the ribosome, a structure that translates detailed instructions to create proteins. Despite the fact that ribosomes across all cells are quite similar, they’re picky about how they receive instructions. They are also wildly inefficient.
Cells leverage DNA to store instructions and a uniform alphabet and language to decode, decipher, and build from them. Amino acids function much like alphabetical letters do. Stringing enough of them together results in proteins (words), protein complexes (sentences), and ultimately complex biological activities (paragraphs).
The “alphabet” of amino acids as we know it is just half the story, though. Each amino acid comes in two shapes, which are mirror images of each other—a right-hand version and left-hand version. In biology, proteins are composed of only left-handed amino acids, which leaves the right-handed protein world largely unexplored.
This has long puzzled scientists, including Adamala. When thinking about developing a synthetic cell system, Adamala wondered if she could leverage right-handed amino acids, in part because down the road they show promise as therapeutics. She’s also motivated to learn whether life could exist in a “right-handed” form. This concept grabbed the attention of Evan Kalb when he was just starting his graduate program. Now a fourth-year Ph.D. student in Adamala’s lab, Kalb spends a lot of time thinking about how to create more “flexible” ribosomes.
“We are not trying to change the chemical functionality of the proteins. We are just trying to change their shape,” says Kalb. This will come as a relief to biochemists who know firsthand that biology is already chock-full of complex chemistry, much of which is still not explained.
One key application is utilizing mirrored amino acids in pharmaceuticals, both in outer space and closer to home. Protein therapies debuted in the 1920s with insulin and involve introducing small proteins into a patient’s body. The therapy is used to ease pain, replace hormones, and speed up injury recovery timelines. While some of these small proteins will make it to their target, many are chopped up before they do. A suite of enzymes known as proteases do a great job of breaking down foreign proteins. Proteins containing mirrored amino acids are harder for these proteases to recognize and thus show promise in developing more effective protein therapies.
While Kalb is driven by the potential applications, he also frequently ponders the philosophical question, “What would a mirror life look like?”
While individual amino acids don’t change their function when right- or left-handed, does that hold true if scaled up to a living, breathing organism? While this “right-handed” organism is a long way from its debut, the prospect keeps researchers intrigued.
Oily alternatives
Drug development is just one of several game-changing applications. Another includes alternative fuels that could dramatically reduce our dependence on fossil fuels. Chemical compounds derived from petroleum—known as petrochemicals—make up a massive number of products on consumer shelves (not to mention the shelf itself!). Plastics, coatings, gels, and fertilizers all use petrochemicals.
Technologies supporting the expansion of wind, solar, and hydrogen are expanding rapidly, but alternatives to petrochemicals remain stubbornly out of reach. Chemists working to develop them at the bench have come up short time and time again. In part, this is because biological processes can produce chemicals so complex that when a chemist goes to synthesize them at the bench, it’s exceedingly expensive and difficult.
Over the years, researchers successfully coaxed bacteria and cell systems to produce a range of common chemicals, including ethanol, butanol, and vinegar. They also succeeded in crafting more complex chemicals, including precursors to morphine and a drug to treat malaria. No such luck with petrochemicals.
“Petrochemicals are bad for anything with an ounce of common sense,” says Adamala. “No bacteria will make them because it will kill them.” Chemicals like ammonia and benzene come from crude oil and are toxic to cells.
Since synthetic cell systems don’t have the same self-preservation tendencies of natural cells, they show promise as biologically viable chemical factories.
Building together
Decade-old rivalries between lab groups often drive discord and conflict amongst researchers. The emerging field of synthetic biology is unusually collaborative, in part because it is only now becoming established. Adamala saw an opportunity to bring the community together with an eye to sharing successes and failures. And thus emerged the Build-A-Cell Network.
“When a synthetic cell is developed, it will be one of the biggest discoveries in the history of science. That isn’t the accomplishment of a single lab or even a single country,” says Adamala. “It should belong to the scientific community.”
This collaborative mindset means sharing failed experiments with colleagues. For Ph.D. students, that can mean gaining years of their life back. Working in a collaborative field saved a lot of headaches and time for Nathaniel Gaut, a member of Adamala’s lab who recently defended his dissertation. For one of his key projects, he worked with a piece of technology that was finicky. After connecting with a colleague in another lab, he learned they also really struggled to get it to work reliably under controlled conditions. Knowing this, Gaut pivoted his approach, which saved months of frustration down a dead-end path.
“It’s really powerful to not have the field repeat stuff over and over again if it doesn’t work. Letting people know, we tried this, and it didn’t work, saves people time,” says Gaut. “It can make a field move much more rapidly because then they can go on and do something else that actually hasn’t been done before.”
Scientific journals don’t publish “unsuccessful” experiments. If a researcher cannot get a new method to work, they have lab mates to help lift their spirits. But often that’s where tales of failure ends. Another researcher half-way around the world might attempt the same tactic—unbeknownst to them that it’s a prickly path.
Different research groups are focusing on different aspects of the system. Adamala’s research group is one of the groups that’s hoping to bring together multiple pieces into a synthetic cell.
Fuzzy finish line
The first footprints on the moon, the first successful administration of a vaccine, and the first flicker of the electric light bulb all have something in common. They are all lines on a lengthy list of groundbreaking scientific achievements. They all also had a clear finish line.
Synthetic cell systems are poised to join the ranks in the coming years, but the finish line isn’t as obvious.
These synthetic cells will divide, create proteins, and discard waste, to name a few functions. They’ll resemble a pared-down version of a cell. Initially, the synthetic cells will require a very specific “diet” in order to survive. Akin to early computers that took over multiple stories compared to the smartphone in our pockets, early synthetic cells might be unrecognizable in another fifty years.
“We’ve never seen a living to nonliving transition happen before our eyes. Nothing has ever become alive that wasn’t alive before,” says Adamala. “That’s one major reason that defining a finish line is so challenging.”
Despite the finish line being a little fuzzy, Adamala is optimistic about seeing a synthetic cell system in her lifetime. “The science is just moving forward so incredibly fast, that there are things we didn’t even imagine would be possible 20 years ago, and now they’re routine.” At least for a while, spaceships will need fully stocked medicine cabinets. —Claire Wilson